Electron Backscattering Patterns and Ion Blocking Patterns
Automated
Crystal Orientation Microscopy (ACOM), or in short Orientation
Microscopy (OM), on bulk surfaces is usually based on Backscatter
Kikuchi Diffraction (BKD) in the SEM. A commercial acronym for this
technique is EBSD. Spatial resolution is usually in the range of 0.05
μm, accuracy is better than 0.5°, and speed of on-line measurement is
actually more than 1000 patterns per second with Fast EBSD. An
alternative technique yielding higher spatial resolution is microbeam
electron diffraction in the TEM or SEM using spot (SAD) or transmission
Kikuchi patterns (TKD). Thin foils, however, are difficult to prepare
for observation in transmission and, even worse, are sources of
orientation errors due to wrinkling which is frequently manifested by
bend contours. The study of fine grain and heavily deformed materials
in general, of nanomaterials, of recrystallization, grain growth and
the characterization of grain boundaries demand for a substantially
higher spatial resolution than is achieved with EBSD in the SEM.
1. Introduction to Ion
Blocking Patterns
Diffraction
patterns can be produced not only by electrons, but as well by ions of
some ten keV kinetic energy when impinging on a crystalline surface.
The first Ion Blocking Patterns (IBP) have been published by A.F.
Tulinov in 1965 [1, 2]. The patterns
have been produced by proton beams of 150, 200, and 500 keV steered onto
a single crystal of W respectively Mo at an angle of incidence of 30°.
They have been recorded on special photographic plates, placed parallel
with the surface of the crystal, to cover a large solid angle. Patterns have been indexed in these papers and in
further papers published in the same year by A.F. Tulinov and
co-workers, so providing evidence of the crystallographic nature of the
patterns and of the mechanism of their generation by channeling the
ions along low-index lattice planes and crystallographic directions.
Backscatter Kikuchi Pattern from Cu at 20 keV
|
Proton blocking pattern from W at 200 keV [1].
The permission for
reproduction is gratefully acknowledged to Prof. A.F. Tulinov, Lomonosow State University
Moscow, and Uspekhi Fizicheskikh
Nauk, Moscow.
|
IBP
have, at a first glimpse, an appearance substantially different from
Backscatter Kikuchi Patterns (BKP):
1. Instead of broad Kikuchi
bands, they show narrow straight bands of high contrast, nearly black
lines, due to the shorter deBroglie's wavelength of ions. Background is more even and continuous. Band profiles are
described by almost symmetrical cusps.
2. The band width is given by
the critical channeling angle rather than by the Bragg angle.
3. The band widths also depend
on the atomic number of the target atoms of the crystal. This is not
the case in electron diffraction.
4. No high-order lines are seen.
High-order Kikuchi lines are very useful in the precise determination
of orientation and lattice parameters.
5. Dark patches mark low-index
zone axes.
6. Intensity is high also in
backward scattering direction whereas BKP have a strong intensity
maximum in forward scattering direction.
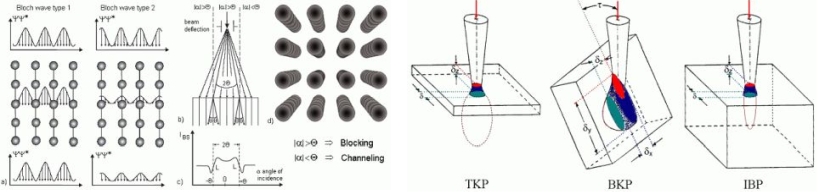
Simple models of generation of BKP by channeling and blocking
|
Interaction volumes of TKP, BKP and
IBP
The probe size is marked in red, and spatial resolution in blue.
|
Kikuchi
patterns are clearly generated by diffraction, whereas IBP could
perhaps be better interpreted by the classical ballistic model of
channeling. Precise experimental measurements are still missing so as
to decide whether Bragg's equation is valid here. The background is
produced by blocked ions according to Rutherford scattering. There is
no significant difference in backscattering from an amorphous and from
a crystalline solid for ions that impinge at directions off the
critical angles (~ Bragg angles). At kinetic energies in the range of several ten keV,
their mean free paths are less than a few atomic layers. Only the
channeled ions are missing in case of a crystal, they penetrate deep in
the crystal and form sharp cusps of low intensity on the background. So
the background intensity can be used as signal. This information about
the crystal structure comes from the upmost atomic layers, like Auger
electrons. As a consequence, spatial resolution in IBP is only limited
by the diameter of the primary beam probe. On the other hand, BKP are
formed by electrons that are backscattered from the interaction volume
that extends beneath the surface to a depth of the mean free path of
energetic electrons. Hence spatial resolution in EBSD is limited to
some ten nanometer, despite that the electron beam can easily be
focused to a significantly smaller probe size.
The geometrical basics of IBP and BKP, however,
are quite similar. The center line of a band corresponds to the section
line of the - imaginarily extended - diffracting lattice plane with the
recording plane. The crossing points of bands, named poles,
represent the positions of zone axes of the crystal. So the crystal
structure and crystal orientation of the diffracting volume can be
determined from the intensity distribution and positions of the bands
in an IBP in quite a similar way as in a BKP. As an application, C.S.
Barrett and co-workers [3] have used proton blocking patterns at 100
keV for phase differentiation. Crystallographic aspects of ion beam
scattering have been discussed in a review article [4]. A commercial
instrument for the identification and rapid alignment of single
crystals was manufactured by Edwards High Vacuum Intern. [5].
________
[1]
A.F. Tulinov: On an effect accompanying nuclear reactions in single
crystals and its use in various physical investigations. Soviet Physics
- Doklady 10 (1965) 463-465 (English translation of the original
article of A.F. Tulinov, Doklady Akademii Nauk SSSR 162 (1965) 546-548)
[2] A.F: Tulinov, V.S.
Kulikauskas and M.M. Malov: Proton scattering from a tungsten single
crystal. Physics Letters 18 (1965) 304-307
[3]
C.S. Barrett, R.M. Mueller and W. White: Proton blocking patterns for
hcp and wurtzite structures. Transactions MS AIME 245 (1969)427-429
C.S. Barrett: Line intensities
in proton scattering. Transactions MS AIME 245 (1969) 429-430
[4] C.S. Barrett: Ion Beam
Scattering applied to crystallography. Naturwissenschaften 57 (1970)
287-295
[5] R.G. Livesey: A 30 keV
instrument for ion surface interactions studies. Vacuum 22 (1972)
595-597.
2. Orientation Microscopy
in the Ion Scanning Microscope
The
working principles of ion scanning microscopes and of the more common
Scanning Electron Microscopes (SEM) are very similar, with the
exception of an ion gun and electrostatic or quadrupol lenses which
replace the electron gun and round magnetic lenses. Ion scanning
microscopes are used in the microelectronic industry to repair masks
and electronic circuits. Focused Ion Beam (FIB) appliances to the SEM
or stand-alone ion scanning microscopes with a Liquid Metal Ion Source
(LMIS) ( Ga+ ) are frequently employed in materials science for the
preparation of TEM and SEM specimens by ion milling. Bulk surfaces are
imaged in scanning mode whereby ion induced electrons, backscattered
primary ions, secondary ions which have been released from the sample,
neutrals or cathodoluminescense radiation are used as signals.
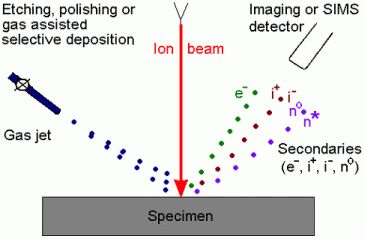 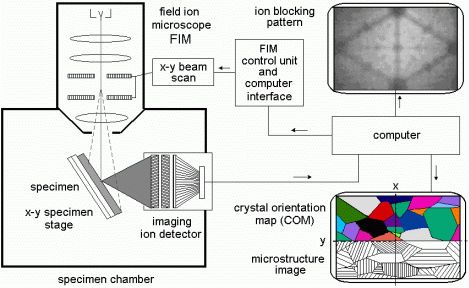 |
|
A
similar technique as for BKD has been proposed for the acquisition and
indexing of Ion Blocking Patterns (IBP) in an ion scanning microscope
[2]. Orientation microscopy with IBP promises the following advantages
over EBSD in the SEM:
• The sample is tilted at
moderate angles of about 45° to the primary beam direction to
accommodate the wide-angle pick-up of IBP. So image distortion and
spatial resolution in beam direction are markedly reduced as compared
to EBSD where the specimen is steeply tilted to typically 70°.
• Sample preparation is
less difficult since deformation layers or foreign surface layers can
be removed in situ at a controlled rate by using a primary beam of
heavy ions until clear blocking patterns have developed.
• 3D reconstruction of the
volumetric microstructure from planar 2D slices is facilitated by
controlled serial ion sectioning since the specimen may remain
stationary in this position or is merely tilted to a steeper angle to
the beam. The significantly smaller information depth of a few atomic
layers favors 3D reconstruction.
• Specimen charging is
less harmful than in the SEM because secondary electrons are released
from walls of the specimen chamber by the impact of scattered ions and
neutrals. They reduce positive surface charging.
The combination of ACOM/IBP with a Helium Ion Microscope Zeiss Orion®
[3] promises particular advantages:
• Virtually no specimen
sputtering is induced by the light-weight Helium ions during
orientation measurement nor during imaging the microstructure.
• The excitation volume is
not significantly larger than the minimum spot size. Spatial and
in-depth resolution of ACOM with IBP is expected to approach the
sub-nanometer range.
• Specimens that adversely react
to Ga+ ions, such as aluminum base alloys, can be investigated.
However, beam current in small ion probes is presently very low in the Orion®
Ion Scanning Microscope, so that we did not yet succeed in producing
sequences of IBP. A more feasible alternative may be a stand-alone FIB
ion microscope with a Ga+ LMIS, but to the disadvantage of less spatial
resolution.
The blocking-and-channeling effect is manifested
in an exceptionally high orientation contrast when a parallel beam of
energetic ions is scanned across a polycrystalline surface. Small
angular tilts of the specimen lead to strong changes of contrast
between the grains [1]. This is an indication of the pronounced
anisotropy of ion backscattering from crystals, an effect which is
clearly reflected as well in the polar intensity display of the 2D IBP.
Orientation contrast as a function of specimen tilt in the field ion
microscope.
Copper specimen imaged with electrons induced by impact of Ga ions at
30 keV in the Ion Scanning Microscope.
I thank Prof. Dr. U. Wendt, University of Magdeburg, Germany, for
providing this figure.
|
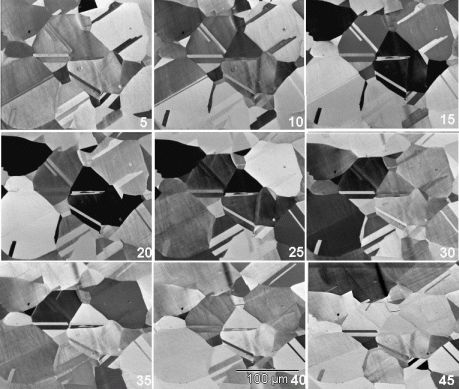
|
________
[1] U. Wendt and G. Nolze: FIB
milling and channeling. G.I.T. Imaging & Microscopy 9#3 (2007) 34-36
[2] R.A. Schwarzer: Spatial
resolution in ACOM - What will come after EBSD. Microscopy Today 16 #1
(2008) 34-37
[3] L. Scipioni, L. Stern and J.
Notte: Applications of the Helium Ion Microscope. Microscopy Today 15
#6 (2007) 12-15
|